This paper shows how organic carbon, when deeply buried and transformed into graphite, can survive multiple cycles of erosion, transport and burial. It is available, open-access, from the journal website.
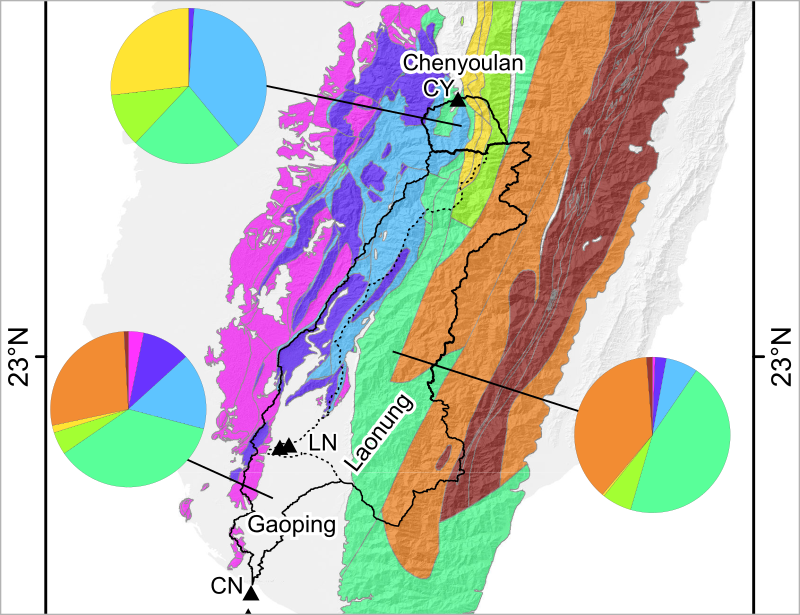
The samples came from Taiwan, which has a pretty extreme tectonic and climatic setting. The convergence of the Eurasian and Philippine Sea plates leads to rapid mountain building, and the impact of severe typhoons each year leads to large amounts of erosion. This means that lots of sediment is removed from the island each year, including from rocks that were previously buried deep under the island, and metamorphosed. I looked at samples collected from several river catchments in the southwestern part of the island, and from some offshore cores. Some of these catchments drained the Central Range mountains, and others the Western Foothills and Coastal Plain. The rocks in these two regions are very different, especially in terms of the carbon they contain.

The analytical work was based around the Raman spectroscopy technique I developed during my PhD (published back in 2013). Raman spectra were collected from particles of organic carbon in the sediments, and automatically processed to determine which Raman peaks were present, where they were located, how tall and how wide they were. The peaks found in each sample were used to show the types of carbon present in each rock. As each particle is analysed independently, there is no averaging effect if a samples is a mixture of several sources.
This analysis showed that rivers draining the youngest rocks, which had experienced the least metamorphism, had the most graphite in. The rivers draining the most metamorphic rocks had little or no graphite. This could only be explained if the graphite was eroded from somewhere else and then deposited into the Taiwanese sediments before they became rocks. The graphite-rich rocks were sourced from Taiwan itself – the rapid tectonic uplift means that a lot of material has been removed from the top of the mountains and washed into the surrounding ocean. Yet there are no rocks in Tawain that have been buried deep enough to make graphite, so the original graphite must have come from somewhere else entirely!

Our best guess is that China was the original graphite source, since there are lots of graphite bearing outcrops in the regions near to Taiwan, eroding into the South China Sea. So the first phase of recycling was from China out onto the ocean floor before Taiwan became an island. Once the island appeared out of the ocean, the second phase of erosion moved these graphite rich sediments from the newly formed land back into the nearby seas (the second recycling phase). These rocks were then uplifted themselves, forming the Western Foothills of the island, and are now eroding for the third time out into the South China Sea. During all this time, some of the graphite has survived, and can be seen easily in the modern sediment.
All this means that graphite crystals are pretty stable, and can survive being eroded, transported and buried in sediments multiple times. They can be used as tracers, because although the rock they came from has been broken up into tiny pieces and dispersed across the ocean floor, each graphite flake can be characterised very precisely by Raman.
Turning organic carbon into graphite also gives it stability, stopping it from degrading back into carbon dioxide. On long timescales, this means that carbon is transferred from the atmosphere into the biosphere (trees and plants), and then into the lithosphere (rocks) where it can survive for millions of years.